Kenneth Johnson
- Professor
- Roger J. Williams Centennial Professorship in Biochemistry
- Molecular Biosciences
- Interdisciplinary Life Sciences Graduate Programs
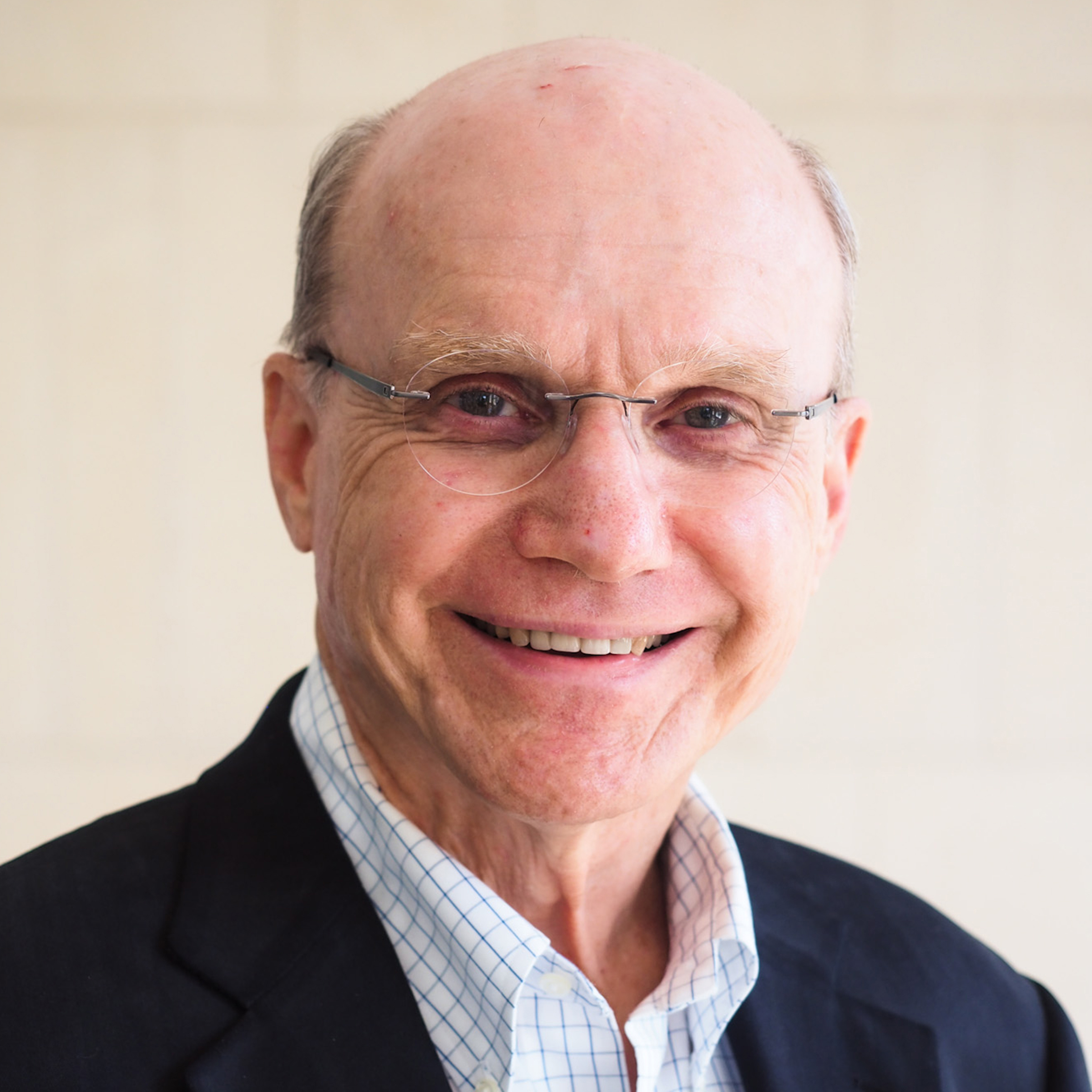
Contact Information
Biography
Dr. Johnson received his Ph.D. in 1975 in Molecular Biology at the University of Wisconsin and completed postdoctoral studies at the University of Chicago with Edwin W. Taylor where he learned transient kinetic methods. He joined the University of Texas at Austin in 1998 after having served on the faculty of Penn State University as the Paul Berg Professor of Biochemistry. He is currently the Roger J. Williams Centennial Professor of Biochemistry as a member of the Department of Chemistry of Biochemistry and of the Institute for Cell and Molecular Biology. He is the recipient of the Pfizer Award in Enzyme Chemistry, the Penn State Faculty Scholar Medal and is a member of the Editorial Board of the Journal of Biological Chemistry. He has designed and built instruments for transient kinetic analysis and made them available through his company, KinTek Corporation that he founded in 1987. He has established the mechanistic basis for DNA polymerase selectivity during polymerization using modern kinetic methods that he developed. His recent work has shown that the toxicity of nucleoside analogs used to treat AIDS is correlated with their rates of incorporation by the mitochondrial DNA polymerase.
Research
Research in the Johnson Lab is currently focused on four topics. In each we apply state-of-the art kinetic methods developed in the Johnson lab to answer mechanistic questions of biological importance
1. Effectiveness versus toxicity of nucleoside analogs used to treat HIV infections and the mechanistic basis for the evolution of resistance. In our prior work we were the first to establish a quantitative link between the toxicity of nucleoside analogs and the kinetics of incorporation by the human mitochondrial DNA polymerase. We also were the first to quantify the kinetics of incorporation of normal nucleotides and nucleoside analogs by HIV reverse transcriptase. This enabled us to compute an enzymatic toxicity index defining the effectiveness versus toxicity of nucleoside analogs based upon their kinetics of incorporation by HIV RT versus the human mitochondrial DNA polymerase, respectively. We are continuing this work by developing new methods to measure the steps leading to the incorporation of nucleotides on the millisecond time scale and to apply these methods to understand the evolution of resistance to nucleoside analogs.
2. Role of protein conformational dynamics in enzyme specificity. The role of induced-fit in enzyme specificity has long been debated in the enzymology field. In our recent work on HIV RT and on other DNA polymerases we have established the role of substrate-induced changes in enzyme structure in enzyme specificity. Binding of the substrate in an initial weak-binding state triggers a large conformational change with movements up to 25 Å, to hold the substrate tightly and bring catalytic residues into proper alignment to facilitate catalysis. In contrast, binding of an incorrect substrate leads to weak binding and misalignment of catalytic residues. Thus the large conformational change, occurring on a millisecond time scale, determines enzyme specificity. Further studies to understand the kinetic and thermodynamic basis for enzyme specificity are based several upon novel approaches including single molecule kinetic methods to monitor events occurring at the active sites of individual enzymes, and molecular dynamics simulations to predict the pathway and energetics of the conformation change.
3. Structure and mechanism of the human mitochondrial DNA helicase. Replication of the human mitochondrial DNA requires the cooperative action of the DNA helicase to unwind the DNA and the DNA polymerase to replicate each single strand. The DNA helicase forms a hexameric ring around single stranded DNA and, in a reaction coupled to ATP hydrolysis, walks along the single stranded DNA to displace the duplex. We are currently working to reconstitute the replication complex and study the role of ATP binding and hydrolysis in DNA unwinding.
4. Correlating mutations in the human mitochondrial DNA polymerase and helicase with heritable diseases. There are numerous mutations in the humane genes for the mitochondrial DNA polymerase and helicase that are linked to heritable mitochondrial disorders. We are using our sophisticated structural and kinetic methods to understand the molecular basis for these defects. Understanding the physiological effects resulting from biochemical defects is challenging and requires the most accurate measurements and comprehensive analysis. To approach this problem and many others, we have developed new computer methods for rigorously fitting kinetic and equilibrium binding data without the standard simplifying assumptions employed in other labs.
Research Areas
- Biochemistry
- Biophysics or Structural Biology
- Molecular Biology or Genetics
- Health Promotion or Disease Prevention
- Infectious Disease, Immunology and Microbiology
Fields of Interest
- Structural Biology and Biophysics
- Biochemistry
- Molecular Biology, Genetics & Genomics
Centers and Institutes
- Interdisciplinary Life Sciences Graduate Programs
Education
- Ph.D., Molecular Biology, University of Wisconsin (1975)
- B.S., Chemistry, University of Iowa (1971)